The mechanism of action of 4-MMC in terms of its interaction with monoamine transporters is widely studied and fairly well described, but despite this, information on the involvement of glutamatergic transmission with respect to the effects of mephedrone is still limited. In the mammalian brain, glutamate is the predominant excitatory neurotransmitter, functioning through a variety of receptors that may be split into two main groups: ionotropic glutamate receptors (iGluRs, fast-acting ligand gated ion channels) and metabotropic glutamate receptors (mGluRs, slow-acting G-protein-coupled receptors). Group of iGluRs consists of N-methyl-d-aspartate (NMDA), α-amino-3-hydroxy-5-methyl-4-isoxazole propionic acid (AMPA) and kainate receptors. Eight mGluR subtypes (mGluR1–mGluR8) have been discovered so far; these subtypes may be grouped into three categories based on their pharmacological selectivity and signaling pathways.
The main purpose of the present study is to evaluate and analyze the role of glutamatergic transmission in the rewarding effects of mephedrone, given the strong evidence linking glutamate to addiction and pleasure. This objective was accomplished by behavioral research, in vivo imaging methods, and ex vivo biochemical analyses. For the behavioral tests, memantine, a non-competitive glutamatergic receptor antagonist, was evaluated for its effects on the expression of mephedrone-induced CPP using a well-established paradigm of conditioned place preference (CPP). Given the fact that data on the quantification of glutamate in specific brain zones after mephedrone administration are few and often contradictory, the next step of the study was to assess glutamate levels, both in vivo and ex vivo, in the hippocampus, which is, along with the mesocorticolimbic reward system, one of the major structures associated with the emergence of addiction.
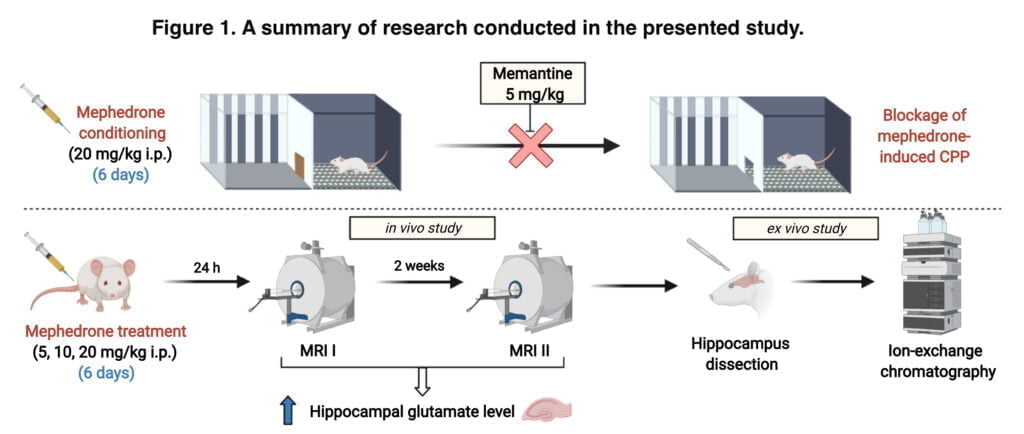
We combined two complementing techniques (ex vivo and in vivo). First, after administering mephedrone for six days, in vivo magnetic resonance spectroscopy was used to detect the hippocampus glutamate level changes that were time- and dose-dependent. However, to prove the validity of this method for further usage in behavioural research, we verified MRS measurements with the ion-exchange chromatographic method and performed ex vivo biochemical assessment of glutamate hippocampal levels in rats subjected to two MRS measurements after 6 days of mephedrone administration. Together, the data presented offer a sophisticated and thorough understanding of the role glutamatergic activity plays in the rewarding effects of mephedrone. Figure 1 displays the research’s experimental setup for the presented study.
Experiment design methodology
In our study, we used rats at 8 weeks of age at 200-250 grams. Mephedrone hydrochloride and memantine hydrochloride were used as main substances. We administered the first substance at a dose of 20 mg/kg for six consecutive days, and memantine was administered at a dose of 2.5 mg/kg and 5 mg/kg once 30 minutes before testing for mephedrone-induced CPP severity. A dose of 2 ml/kg of medication was injected intraperitoneally. Saline solutions (0.9% NaCl) were administered to control groups using the same method and dose as experimental groups. Every day of the experiment, the solutions were produced from scratch. Preliminary research indicated that mephedrone at a dosage of 20 mg/kg produced the strongest response in the CPP paradigm, therefore this dose was chosen to assess the role of glutamatergic systems in the rewarding qualities of mephedrone in the current study. Rats were given anesthesia for the MRI research using a 3.5% isoflurane and 100% oxygen mixture at a flow rate of 0.7 l/min.
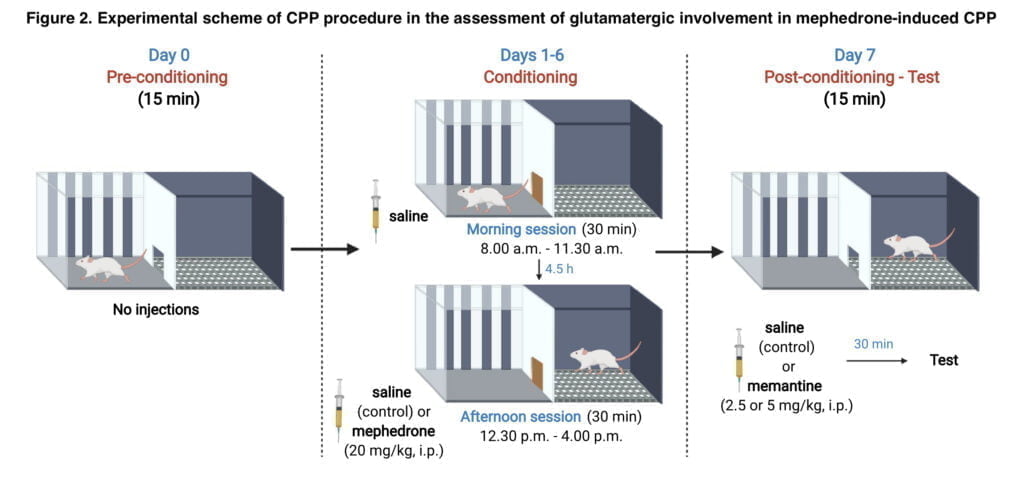
CPP Procedure CPP consisted of 3 phases: pre-condition- ing, conditioning and post-conditioning. To reduce stress that can alter the behavioural response, animals were acclimated to the apparatus for 15 minutes the day before the CPP procedure. The guillotine door was left open on day 0 of pre-conditioning, allowing animals full access to both compartments. The guillotine door was shut on days 1-6. Each animal was kept in a separate compartment during the morning session. The saline injection was given to the animals in the morning session. Animals received injections of saline or mephedrone (20 mg/kg) right before the afternoon session. Morning and afternoon sessions were separated by a 4-h interval. In day 7, saline or memantine (2.5 mg/kg or 5 mg/kg) was administered 30 min prior to the testing. The entrance to the guillotine was open. Both compartments were freely accessible to the animals, and post-conditioning preference was assessed. Figure 2 displays the experimental CPP method plan.
Locomotor activity was also assessed due to the possibility that an animal’s motion may affect the CPP results. Since the goal of our study was to assess whether administered drugs affected animals’ locomotion during post-conditioning test, total horizontal activity was recorded for 15 min on the test day. An evident benefit of this approach is the ability to assess CPP values and locomotor activity levels simultaneously without placing the animals under additional stress. For the MR investigation, 36 rats were split into 4 study groups, each of which received saline or mephedrone at doses of 5 mg/kg, 10 mg/kg, or 20 mg/kg for 6 days straight before to the first examination. Warm water in a closed circuit was used to confirm that the body temperature was kept at roughly 37 °C. After the second MRI measurement, animals were decapitated and the whole brain was carefully taken out and rinsed in isotonic saline to remove blood. To evaluate glutamate concentration, hippocampi were homogenised and deproteinised in 6% sulphosalicylic acid in lithium citrate buffer in 1:10 ratio. Chromatograms were evaluated for glutamate concentration.
The results of our study and discussion
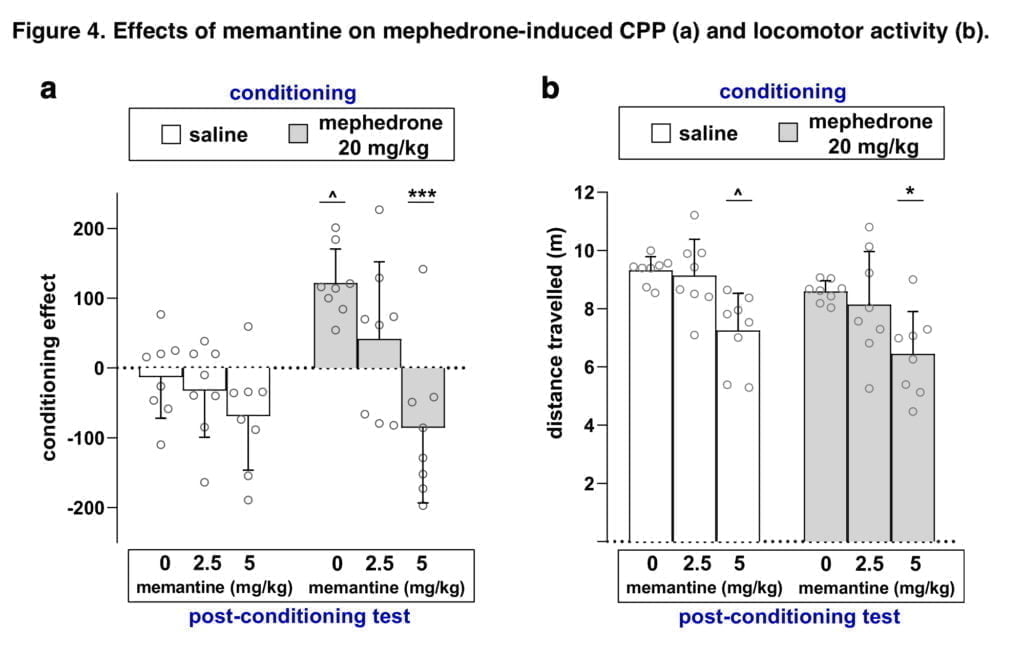
The CPP test was used to evaluate the rewarding effects of mephedrone as well as the part glutamatergic neurotransmission plays in the manifestation of these effects. In the research that was presented, mephedrone’s rewarding characteristics in the CPP paradigm were validated, and it was paired with an analysis of how much glutamatergic activity contributed to the results that were shown. Most illicit substances interact directly or indirectly with glutamatergic receptors to change glutamatergic transmission in a variety of ways. Additionally, it has been noted that blocking glutamatergic transmission pharmacologically reduces the reinforcing effects of medicines. Mephedrone’s effects on glutamatergic circuits are currently unknown. In this study, we used the drug memantine to assess the contribution of glutamatergic neurotransmission to the manifestation of mephedrone-induced CPP via NMDA receptors. Memantine is an agonist of dopamine D2 receptors and a non-competitive antagonist of NMDA receptors. Also, memantine has the property of inhibiting α-7 nicotinic acetylcholine receptors, affecting 5-hydroxytryptamine and dopamine uptake, and sigma-1 receptors (which are activated by exposure to sodium channels). It has also been discovered that memantine prevented mice from acquiring and recovering from morphine-induced CPP. Memantine also prevented mice from acquiring and reinstating the CPP caused by MDMA.
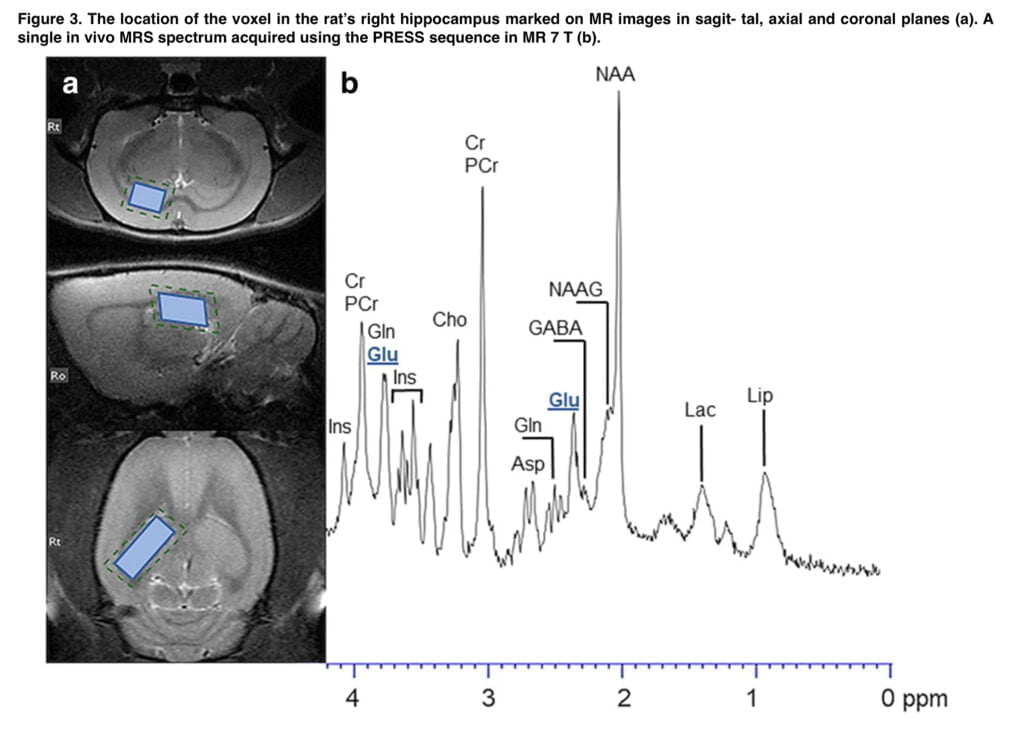
In our study, we observed a significant altered behavioral effect from memantine, which blocked the expression of mephedrone-induced CPP. However, memantine treatment resulted in a reduction in locomotor activity in both the saline- and mephedrone-conditioned groups. This suggests that changes in locomotor activity could have had an impact on the memantine-induced suppression of the rewarding effects of mephedrone. However, given that both the control and mephedrone-treated groups’ mobility was significantly impacted, it may be concluded that memantine’s ability to prevent mephedrone’s ability to elicit CPP rather than the drug’s effect on animals’ mobility is what is likely to be to blame. Furthermore, it should be taken into account that memantine can non-selectively affect different central pathways, e.g. via nAChRs or 5-HT and DA neurotransmission. The study’s limitations that alternative NMDA-independent processes may possibly contribute to the effects of memantine that have been seen are therefore made obvious. Given the conclusive data that demonstrate the ability of memantine to block the rewarding effects of various psychoactive substances, as well as the MRS results showing increased glutamate levels in the hippocampus after mephedrone use, we concluded that glutamatergic neurotransmission is involved in the appearance of CPP induced by mephedrone administration. In order to characterize more specifically the potential participation of other iGluRs and/or mGluRs in the mephedrone-induced expression of drug reward, more study is required. The findings reported here may thus be viewed as a promising basis for that research.
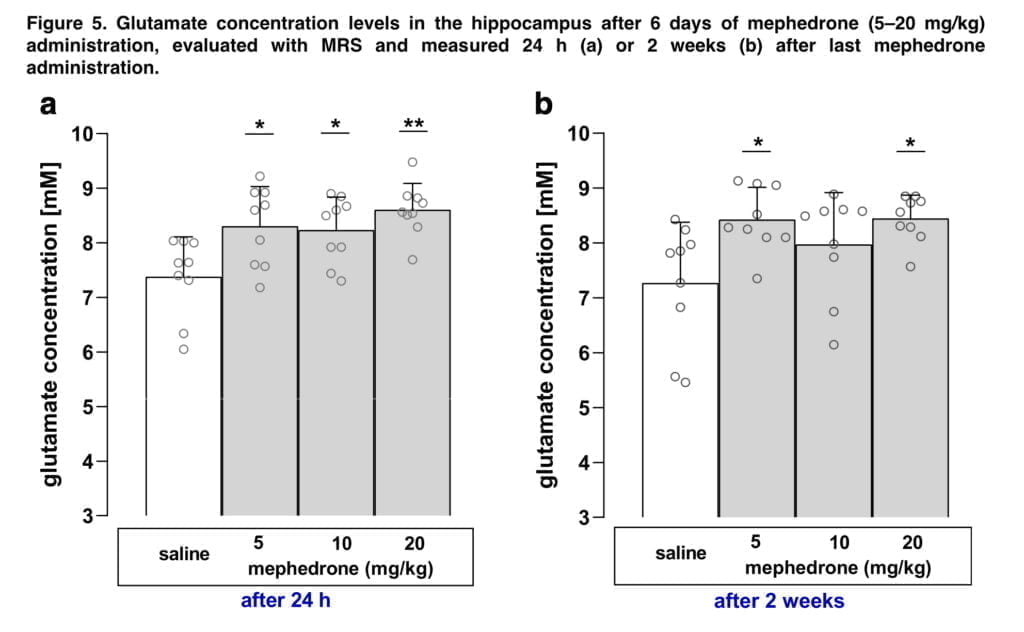
The chromatographic findings are in line with the previously reported ex vivo investigation, which found no significant variations in the hippocampus glutamate levels assessed post mortem in both male and female rats after mephedrone treatment. However, in two time points of measurements, our in vivo MRS measurements were able to identify substantial differences between control and mephedrone-treated mice. The disparity between two approaches may be attributable to physiological variations in the glutamate levels in in vivo and ex vivo tissues as well as the environmental factors that affected the readings. Biochemical results show the content of glutamate in hippocampal tissue after administration of different doses of mephedrone, while the MRS results depict the change or level of this neurotransmitter during brain work (in this case, the work of the hippocampus). Since MRS allows to measure metabolites’ changes in vivo in a non-invasive way, the usage of this technique in behavioural studies has two undeniable advantages. First off, since it doesn’t call for decapitation, the animals can be sacrificed in a less strenuous manner. Second, although ex vivo investigations would require numerous animal groups for repeated measurements at various time periods of observation, MRS allows for the detection of time-dependent changes in the same cohort of animals. As we discovered a rise in glutamate levels two weeks after the final injection of mephedrone, the investigations demonstrated that the modifications in glutamatergic neurotransmission are long-lasting.
Conclusion
The research that is being presented is the first one to use a multidisciplinary method to thoroughly assess glutamatergic participation in the rewarding effects of mephedrone. Utilizing MRS, the behavioral investigations were paired with in vivo imaging of glutamate concentrations in the hippocampus, which was then followed by the chromatographic identification of important metabolites in the animal brains. Overall, the findings offer fresh perspective and reliable proof of glutamatergic participation in the processes linked to mephedrone’s rewarding effects. Also, a comparison of MRI and chromatographic results unequivocally confirmed the validity and importance of the MRS method in neuropsychopharmacological research. Our publication describes a very important study that may serve as the basis for many different studies in neuropsychopharmacology that will look in detail at glutamatergic involvement in the mephedrone-induced manifestation of drug reward in animals.