Various studies have shown that mephedrone significantly increases lipid peroxidation and the expression of antioxidant enzymes (such as superoxide dismutase, catalase, and glutathione peroxidase). Mephedrone treatment in vitro causes a concentration-dependent death of murine neurons. However, mephedrone toxicity to the brain endothelium has not been addressed yet. The blood-brain barrier (BBB) is essential for the function of neuronal networks, and popular drugs of abuse negatively impact BBB integrity and cause endothelial activation. Methamphetamine and MDMA, well characterized drugs of abuse with close structural and mechanistic similarities to mephedrone, were both reported to disrupt the BBB in vivo as evidenced by brain edema and leakage of Evans blue dye in the brain parenchyma. In vitro, methamphetamine treatment results in dose-dependent decrease in transendothelial electrical resistance and increased passage of fluorescein isothiocyanate (FITC)-labeled dextran. Loss of BBB integrity is often attributed to the abnormalities of tight junctions and neuroinflammation.
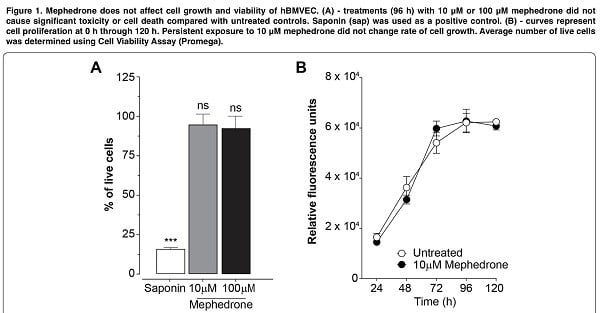
Methamphetamine use results in decreased expression of zona occludens 1, occludin, and claudin-5 both in vivo and in vitro, activation of MMP-9, and increased secretion of proinflammatory cytokines. In addition, the main pathological characteristic of the acute toxicity of mephedrone and methamphetamine is cerebral edema, but the mechanism of action of this biological event has not been studied directly. In this publication we explain the effects of mephedrone on the integrity and function of the blood-brain barrier (BBB). In in vitro models of the BBB we will show how mephedrone reduces transendothelial electrical resistance and increases paracellular permeability of the endothelial barrier and demonstrate the results of proinflammatory and angiogenic responses.
What methods and materials were used?
In the experiment, we used primary human brain endothelial cells derived from fetal brain tissue. The LIVE/DEAD viability/cytotoxicity assay (Life Technologies) was used to evaluate mephedrone toxicity to hBMVEC cell. Also, the following methods were used in the experiment:
- Cell proliferation analysis;
- Electric cell-substrate impedance sensing assay – real-time changes of transendothelial electrical resistance were monitored using the electric cell-substrate impedance sensing (ECIS).
- Paracellular permeability assay;
- Quantitative real-time PCR;
- Protein electrophoresis and immunoblotting;
- Angiogenic factors ELISA – to examine the concentration of growth factors secreted by hBMVEC, cells were grown to confluency and treated with 10 μM mephedrone in growth factor-free medium for 24 h;
- MMP9 activity assay – to examine the activity of MMP9 secreted by hBMVEC, cells were grown to confluency and treated with 10 μM mephedrone in growth factor-free medium for 24 h;
- Proinflammatory cytokine expression panel to examine the concentration of proinflammatory cytokines secreted by hBMVEC, cells were grown to confluency and then treated with 10 μM mephedrone in growth factor-free medium for 24 h;
- Nuclear factor kappa B (NF-κB) activation assay – to examine the activation of NF-κB subunits, hBMVEC were grown to confluency, medium was changed to growth factor-free/serum-free medium, and mephedrone was added at 10 μM final concentration for 24 h;
- Immunofluorescence staining – assessment of p65 translocation to the nucleus was performed by indirect immunofluorescence;
- Flow cytometry – cells were washed with calcium- and magnesium-free PBS, detached using accutase and then pelleted by centrifugation at 1000 rpm for 5 min.
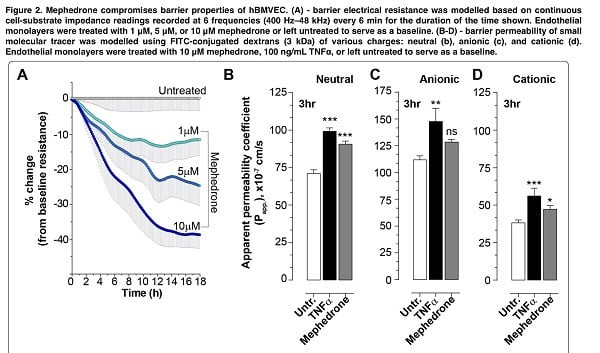
The results obtained and an explanation
Our experiment began with an attempt to evaluate the effect of mephedrone on cell viability. Thus, mephedrone concentrations up to 200 μM did not cause significant cell death in the hCMEC/D3 line we obtained. At the same time, in other studies, MDVP (methylenedioxypyrovalerone) at such concentrations already reduced proliferation and increased necrotic cell death in primary bovine culture. However, such results can be explained in different interpretation and extrapolation of the metric system for calculating concentrations.
The second step was to study the effect of mephedrone on barrier function. In order to assess barrier integrity, we measured electrical resistance and paracellular permeability of the hBMVECs cell family that had been treated with mephedrone. Already after the first mephedrone treatment, a dose-dependent decrease in the electrical resistance of the barrier was detected in the endothelial monolayers, and for 10 μM mephedrone about 40% of the basal resistance was lost during the first 12 hours. Interestingly, all concentrations of mephedrone evoked the same temporal pattern with most of the loss in resistance occurring during the first 12 h post-insult, and the degree of the reduced resistance was dose dependent. We also found that mephedrone significantly increases the rate of passive paracellular passage of small molecules. The results suggest that mephedrone has similar effects to methamphetamine, which causes a transient dose-dependent decrease in TEER (based on previous studies).
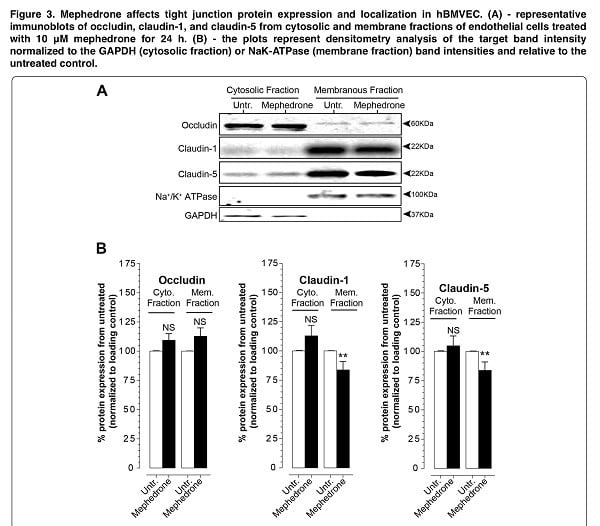
For a more accurate interpretation of the mechanism of mephedrone BBB disruption, we analyzed the expression and subcellular localization of key tight junction proteins that provide the physical part of the blood-brain barrier. Thus, using electrophoresis and immunoblotting, we identified a loss of membrane-bound claudin-1 and claudin-5, which is the cause of the decrease in electrical resistance and increased paracellular leakage we observed (Figure 3). Moreover, mephedrone acts on endothelial expression of claudin-5 both in vivo and in vitro.
The next step was to study the level of subcellular distribution of occludin, which is another key protein of the tight adhesion complex. Occludin is critically involved in the regulation of the blood-brain barrier function in response to cytokines. In the third phase of our experiment, we found that there were no significant changes in the fractions of occludin (Figure 3). Therefore, we hypothesize that mephedrone negatively affects paracellular barrier function specifically through structural changes (claudin-5). It is important to note that intracellular pathways that affect claudin expression/stability do not necessarily affect occludin in a similar way or vice versa. Indeed, although both types of proteins are part of the tight junction complex, their regulation differs. Certainly, these findings warrant further study since it suggests that cathinones initiate divergent intracellular signaling events that affect members of the tight junction complex differently.
When assessing proinflammatory activation of endothelial cells under the influence of mephedrone, we found that mephedrone significantly increases the secretion of the following proinflammatory cytokines: IL-2, IL- 8, IL-13, and TNFα. IL-2 is known to regulate translational modifications of epithelial tight junction proteins and in our results we indeed observed a higher molecular weight of occludin, which is a hyperphosphorylated form.
PECAM-1 is a fairly well studied cell adhesion molecule involved in the regulation of vascular permeability in response to an inflammatory challenge. PECAM-1-mediated vascular wall inflammation is triggered by interleukin 1β and depends on NF-kB activity. When we analyzed the results in our study, we found that extracellular PECAM-1 was increased in hBMVEC as early as two hours after mephedrone use. Thus, increased extracellular localization of PECAM-1 promotes transendothelial migration of effective immune cells in vivo (Figure 4d and Figure 6).
Next, we explored the possibility that mephedrone may be inducing angiogenic program of brain endothelial cells. Surprisingly, we found that mephedrone induces excessive secretion of VEGF-A and PDGF-BB in hBMVEC (figure 5), while cell proliferation kinetics was not affected (figure 1b). Overall, upregulated secretion of angiogenic growth factors, proinflammatory cytokines, and MMPs that we observed in this study resembles the description of inflammaging.
Conclusion
Thus, mephedrone-induced damage to the blood-brain barrier includes the following changes: conversion in the tight junction protein complex, leakage or hyperpermeability of the barrier, vascular endothelial activation, and proliferative inflammation. Given that fatalities in acute mephedrone intoxication are often due to vasogenic cerebral edema, we can conclude that the neuroinflammatory process is the result of impaired cerebral endothelial cell function. Also, in the presence of co-morbidities (e.g., HIV), mephedrone use may contribute to the migration of HIV-infected immune cells across the blood-brain barrier into the brain parenchyma.